What We Review
Introduction
Cells are often called the smallest unit of life. Despite their seemingly tiny size, they display an astonishing array of functions and forms—whether you’re looking at a simple bacterial cell or a highly specialized neuron. But why are cells so small? It all comes down to a key factor: the surface area-to-volume ratio. Understanding why cell size matters and how cells overcome size limitations is essential for grasping fundamental biological concepts and succeeding on the AP® Biology exam.
The Basics of Cell Size
A. Definition of Cells
Cells are the building blocks of life. Every living organism—from single-celled bacteria to complex multicellular organisms—relies on cells to perform life’s essential processes. These processes include metabolism (breaking down and synthesizing molecules), reproduction, and response to environmental changes.
B. Overview of Cell Sizing
Cells come in a wide range of sizes. Most prokaryotic cells, such as bacteria, measure between 1 and 10 micrometers, while eukaryotic cells (like animal and plant cells) are often 10 to 100 micrometers. Given that a micrometer is just one-millionth of a meter, these measurements highlight just how incredibly small cells can be. This small size is crucial because it promotes efficiency in life’s most fundamental processes.
Why are Cells Small? Surface Area-to-Volume Ratio
A. Understanding Surface Area and Volume
Surface area is a measure of how much exposed “outer skin” an object has, while volume measures the space it occupies. For a typical spherical cell:
- Surface Area = 4πr²
- Volume = (4/3)πr³
As a cell grows, its volume increases more quickly than its surface area.
B. Effects of Surface Area-to-Volume Ratios
- Smaller cells have a higher surface area-to-volume ratio, providing more “membrane space” through which nutrients, gases, and waste can pass relative to their overall volume. This higher ratio enables more efficient diffusion, allowing the cell to quickly obtain necessary materials and expel wastes. Conversely, larger cells have a lower ratio, making it harder for materials to move in and out rapidly.
Limitations of Cell Size
A. Constraints on Cell Size and Shape
As a cell’s volume increases, the surface area-to-volume ratio drops, slowing material exchange. Eventually, a cell that grows too large may not bring in enough nutrients or remove enough waste to sustain itself. Cell shapes also play a role: elongated or flattened cells increase surface area relative to volume, thereby mitigating some size constraints.
B. Resource Demand and Waste Management
Larger cells require more energy, oxygen, and nutrients to remain active. They also produce more waste byproducts. If the surface area is too small relative to volume, the cell becomes less efficient at meeting these demands, setting a natural limit on cell size.
Compartmentalization in Eukaryotic Cells
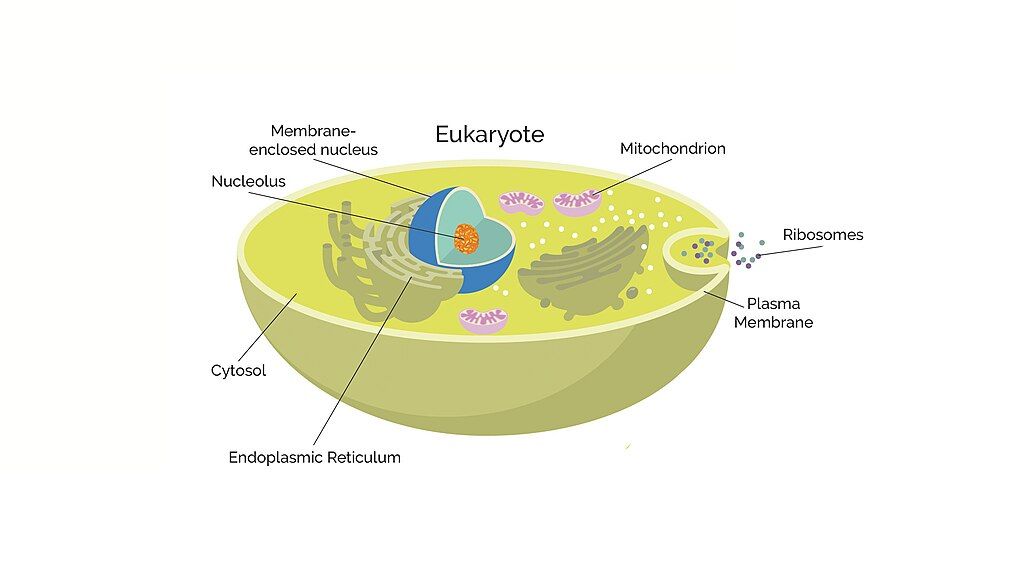
A. Role of Membranes and Organelles
Eukaryotic cells feature internal membranes that form specialized compartments (organelles). This organization allows different processes—like protein synthesis in the rough endoplasmic reticulum or energy production in mitochondria—to occur simultaneously without interfering with one another.
B. Efficient Exchange and Cellular Metabolism
Internal membranes also provide extra surface area for biochemical reactions—for example, the folds inside the mitochondria increase the area available for ATP production. By compartmentalizing various functions, eukaryotic cells keep reactions more efficient despite a relatively larger size than many prokaryotes.
Strategies for Efficient Molecule Exchange
A. Adaptations in Cell Forms
Cells often boast distinctive shapes or structures that help them exchange materials efficiently:
- Microvilli in intestinal epithelial cells drastically increase surface area for absorption.
- Neurons have long, branch-like extensions to transmit signals over greater distances.
B. Examples of Specialized Cells
- Red blood cells (erythrocytes) are flattened and biconcave to maximize oxygen exchange.
- Root hair cells in plants extend long “hairs” for maximum water and nutrient uptake from the soil.
Implications of Size in Larger Organisms
A. Surface Area-to-Volume Ratio in Multicellular Organisms
As organisms grow larger, they face the same issues of exchanging materials efficiently. Organ systems (e.g., respiratory, circulatory) evolve to increase mass flow of oxygen, nutrients, and wastes, compensating for lower surface area-to-volume ratios at the level of individual cells.
B. Evolution of Multicellularity
By becoming multicellular, organisms circumvent the limits imposed by large cell size: many small cells can work together, each maintaining a favorable surface area-to-volume ratio while collectively accomplishing more complex tasks.
Conclusion
A. Recap of Key Points
Cells remain small mainly because a higher surface area-to-volume ratio ensures faster and more efficient exchanges of materials. Eukaryotic cells use compartmentalization to further boost efficiency, and multicellular organisms evolve specialized tissues and organ systems to cope with size increases.
B. Encouragement for Further Learning
Cell size, shape, and compartmentalization are central tenets of biology. Exploring these principles helps us appreciate the intricate balance that sustains life at every level. To deepen your understanding, practice with equations relating surface area and volume, and consider how various cell forms—both in single-celled and multicellular contexts—maintain efficient exchange.
As you prepare for the AP® Biology exam, challenge yourself to calculate surface area-to-volume ratios for hypothetical cells, identify cellular adaptations in various organisms, and reflect on how compartmentalization enables complex cellular functions. By mastering these concepts, you’ll be well-prepared to tackle questions on cell structure, function, and the evolutionary strategies that allow life to thrive.
Sharpen Your Skills for AP® Biology
Are you preparing for the AP® Biology test? We’ve got you covered! Try our review articles designed to help you confidently tackle real-world math problems. You’ll find everything you need to succeed, from quick tips to detailed strategies. Start exploring now!
Need help preparing for your AP® Biology exam?
Albert has hundreds of AP® Biology practice questions, free response, and full-length practice tests to try out.